HYDROGEN STORAGE IN HYDRIDES
Hydrogen storage in metal hydrides
This storage method is based on bonding hydrogen to metal-based materials under convenient temperature and pressure conditions. The reaction of hydrogen with metal is called the hydrogen absorption. Hydrogen is absorbed directly into the material volume. The atomic hydrogen is incorporated into the interstitial space in the crystal lattice of simple crystalline metal hydrides. Hydrogen bonding is an exothermic reaction and heat is released during filling of the tank; therefore, it must be cooled down. During the reverse process when releasing hydrogen, the heat must be supplied externally. Hydrogen is released in the form of gas, and the process can be repeated without any losses of the storage capacity. Reaction thermodynamics, hydrogen adsorption and desorption kinetics, the volumetric and gravimetric capacities, the price, and complexity of the process are investigated. The potential of hydrogen storage in metal hydrides lies in cars and other means of transport use where reversible storage is needed. Hydrogen desorption operates under low temperature and pressure ranges. The optimal operating conditions for fuel cells with polymer membrane are pressure 1–10 atm and temperature 25-120°C. The residual heat of a fuel cell is considered. A simple metal hydride such as LaNi5H6 can be used in this setup. The disadvantages are low gravimetric capacity (cell capacity to cell weight ratio) of hydrogen storage (approximately 1.3 %) and too high expenditure for the automotive industry.
Other materials for hydrogen storage include magnesium-based materials. Their gravimetric capacity is 5-6 % at 260-280°C experimental conditions. Complex hydrides also provide better gravimetric capacity (18%for LiBH4) than simple hydrides, but the reversibility (hydrogen release) is worsened.[1]
Hydrogen storage in chemical hydrides
Hydrogen storage in chemical hydrides is a label for other hydrides that do not contain metals. These hydrides are mainly compounds of boron and nitrogen. Hydrogen is derived by a chemical reaction between hydrides and water or alcohol. This reaction is less reversible than metal hydrides; therefore, its use in vehicles is complicated. The bunt fuel and by-products are withdrawn from the vehicle and for further reprocessing.
Hydrolysis
Hydrolysis is a reaction between chemical hydrides and water to produce hydrogen. One example is a reaction between water and sodium borohydride.
NaBH4 + H2O ⟶ NaBO2 + 4H2
To prevent the reaction (initiated by water) during fuel manipulation, stabilising liquid is used. When the fuel is needed, the stabilising liquid is mixed with water, producing very pure hydrogen. The gravimetric capacity is around 4%. Another material suitable for hydrolysis is MgH2. Its gravimetric capacity in laboratory conditions is up to 11 %.
MgH2 + H2O ⟶ Mg(OH)2 + H2[2]
Hydrogenation/Dehydrogenation Reactions
Hydrogenation is a chemical reaction during which a molecule of hydrogen is added to the compound. The reversed reaction is dehydrogenation, during which hydrogen is released from the compound. Hydrogenation and dehydrogenation as a possibility of hydrogen storage have been studied for many years. One of these reactions is the decalin-to-naphthalene reaction, which can release 7.3% of the mass is released as hydrogen at 210 ºC via the reaction. The advantage of this method is that no water is needed.
C10H18 ⟶ C10H8 + 5H2
New studies focus on the reaction between light metals with methanol or ethanol. Deliberate hydrogen production should be possible at room temperature. The disadvantage is the same as hydrolysis; reprocessing the by-products is complicated. The disadvantage for automotive industry's lies in need for alcohol, which increases the weight and price of the whole process.
The sorption of hydrogen
The investigation of hydrogen storage using sorption has been an important scientific topic past few years. Sorption is a process in which one substance (absorbate) becomes attached to the surface of another (absorbent). The absorption capacity of a substance grows with the size of its surface. Two known methods are physical absorption which uses attraction force, and chemical sorption, which uses chemical bonds.
Carbon Nanotubes
New studies show that the capacity to store hydrogen in carbon nanotubes (CNT) at room temperature and pressure of 8MPa does not exceed the value of 0.42% of mass fraction. CNT bundle storing a large amount of hydrogen in cryogenic conditions. Many theoretical and experimental studies confirmed that hydrogen storage capacity in carbon nanostructures is mediated by weak interaction between H2 and CNT at room temperature. [3],[4]
Boron nitride nanotubes
Another material suitable for storing hydrogen is nanotubes based on carbon and boron nitride (BNNTs). By importing heteroatoms of boron nitride into CNT, interaction of the materials with H2 is stronger than with CNT alone. Studies have shown that the capacity of BNNTS at room temperature is up to 2.6% and can be increased to 4.2% of mass fraction of hydrogen when BNNTs structure collapses.
Pillared graphene
Carbon-based materials have a great potential for hydrogen storage in industry. One of the issues slowing its spread is the storage capacity. It is crucial to increase the number of absorbed hydrogen molecules. It was proved that absorption depends on the material porosity; therefore, a new material (pillared graphene, Fig. 23) was designed. It combines two allotropes of carbon (CNTs and graphene sheets) creating a 3D material with tunable pores.
Small pores in materials prevents hydrogen insertion; large pores, cause residual spaces in the material. Therefore, only ideal-sized pores create efficient hydrogen storage conditions. The variation of pore size can be achieved through the freedom to vary the tube length or diameter, together with the intertube distance.[5]
Overview of alternative technologies
In the previous chapter, the leading alternative hydrogen storage technologies were described. There is a large number of methods under development. In addition, experiments with hydrogen compounds (hydrocarbon, ammonia) are conducted. Currently used methods are improved. Fig.24 shows the principles of conventional and alternative methods.
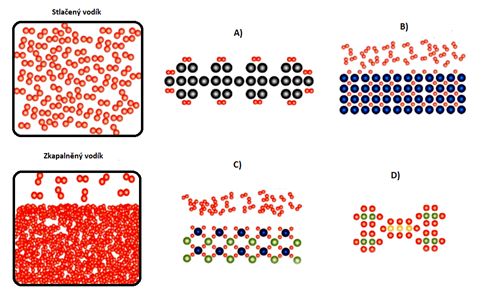
Figures in the first column show compressed and liquified hydrogen. Picture A shows hydrogen storage by absorption on the surface of solid substances, where hydrogen is bound in hydrogen molecules H2 or atoms H. Picture B represents the absorption of hydrogen atoms that are inserted into the material grid (this method shows a large amount of storage in small volumes, at low pressure and almost room temperature). Pictures C and D show complex hydrides, where hydrogen is tightly bound in molecule structures in the form of chemical compounds. The density increases from A to D.[1]
[1] Fuel cell technologies program [online]. Published: January 2011. At http://www1.eere.energy.gov/ hydrogenandfuelcells/ pdfs/fct_h2_storage.pdf
[1] EUROPEAN COMMISSION, Directorate General. Hydrogen storage: state-of-theart and future perspective [online]. Luxembourg: Office for Official Publications of the European Communities, 2003. ISBN 92-894-6950-1.
Available at: http://publications.jrc.ec.europa.eu/repository/bitstream/111111111/6013/1/EUR%2020995% 20EN.pdf
[2] ENERGY.GOV: OFFICE OF ENERGY EFFICIENCY & RENEWABLE ENERGY [online]. Dostupné z: http://energy.gov/eere/fuelcells/fuel-celltechnologies-office
[3] FROUDAKIS, George E. Hydrogen storage in nanotubes & nanostructures. Materialstoday[online]. 2011, vol. 14, issues 7-8. Available at: http://www.sciencedirect.com/science/article/pii/S1369702111701626
[4] Hydrogen storage in nanotubes & nanostructures, online, Available at: https://www.sciencedirect.com/science/article/pii/S1369702111701626
[5] George E. Froudakis, Hydrogen storage in nanotubes & nanostructures,
Materials Today, (https://www.sciencedirect.com/science/article/pii/S1369702111701626)